Evolution of gut bacteria tracks splits in primate species
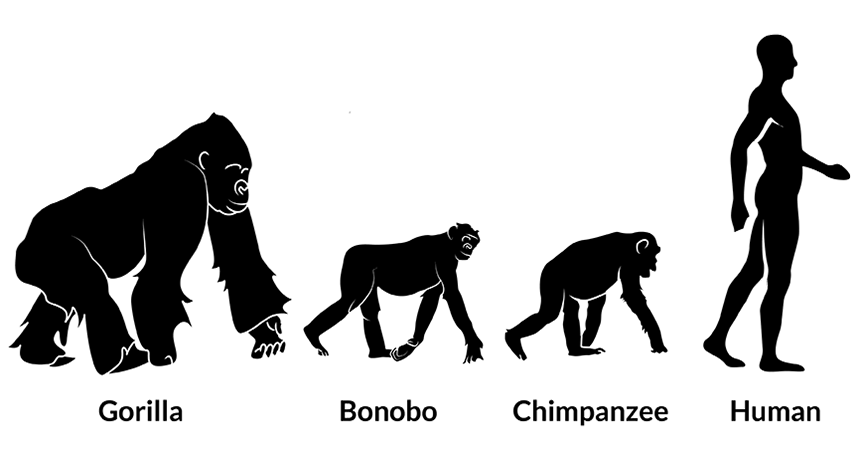
Microbes may have played a role in making us, us. A new study shows similar patterns in the evolution of gut bacteria and the primates they live in, suggesting that germs and apes could have helped shaped one another.
For at least 10 million years, bacteria have been handed down from the common ancestor of humans and African apes. As apes split into separate species, so did the microbes inside them, researchers report July 22 in Science. Now, relationships between gut bacterial species mirror the family tree of gorillas, humans, bonobos and chimpanzees.
Germs are a piece of our history, says evolutionary biologist Andrew Moeller who led the study while at both the University of Texas at Austin and the University of California, Berkeley. “Just like genes we’ve inherited from our ancestors,” he says, “we’ve inherited some of our bacteria from our ancestors as well.”
It’s well known that bacteria are key to human health (SN: 04/02/16, p. 23). They play major roles in the immune system and development. But very few researchers have turned to the past, Moeller says, to ask how humans got those handy bacteria in the first place. His team studied three families of bacteria living in the feces of people from Connecticut, as well as in that of wild chimps, bonobos and gorillas. The scientists used DNA evidence to build relationship trees for each bacterial family, then compared each tree with known relationships between humans and close primate relatives.
Two of three bacterial trees matched primate relationships. For those families, closely related bacteria live in closely related primates. For humans, “the closest relatives of our gut bacteria live in chimpanzees,” Moeller says, “just like our closest relatives are chimps.”
Scientists would expect that pattern to match only if apes and bacteria split into new species in unison. The fact that apes and bacteria split at roughly the same time, while bacteria were living inside of ape species, implies that they were influencing each other, and therefore that the evolution of one group could shape the evolution of the other.
Changing bacteria may have “allowed us to evolve,” says microbial geneticist Julia Segre of the National Human Genome Research Institute in Bethesda, Md., who was not involved in the new work. She and conservationist Nick Salafsky of the nonprofit Foundations of Success, also in Bethesda, wrote a perspective on it in the same issue of Science.
A “very intimate relationship with bacteria,” she says, “is part of who we are.” While the researchers agree that humans and bacteria probably shaped each other’s evolution, they caution that it’s too soon to tell if (and how) ancient apes and microbes changed each other.
Those ancient relationships may get harder to study over time. Industrialization and antibiotics have reduced the diversity of bacteria living in and on humans, Moeller says. And while the microbes in this study have stuck around, other groups may have disappeared or changed dramatically.
One caveat, Segre says, is that humans have been exposed to antibiotics and modern life. Wild African apes might still have their ancient gut flora, but people in Connecticut might not (SN: 12/13/14, p. 10). It’s especially important to do studies like this now, she says, “because it’s not going to get better.”
In the future, Moeller says, researchers should look deeper into the past to see if the gut bacteria living in all mammals share one common ancestor. Scientists could also go the other way, he says, to see if more recently divided human populations also have characteristic gut bacteria.