The classic map of how the human brain manages movement gets an update
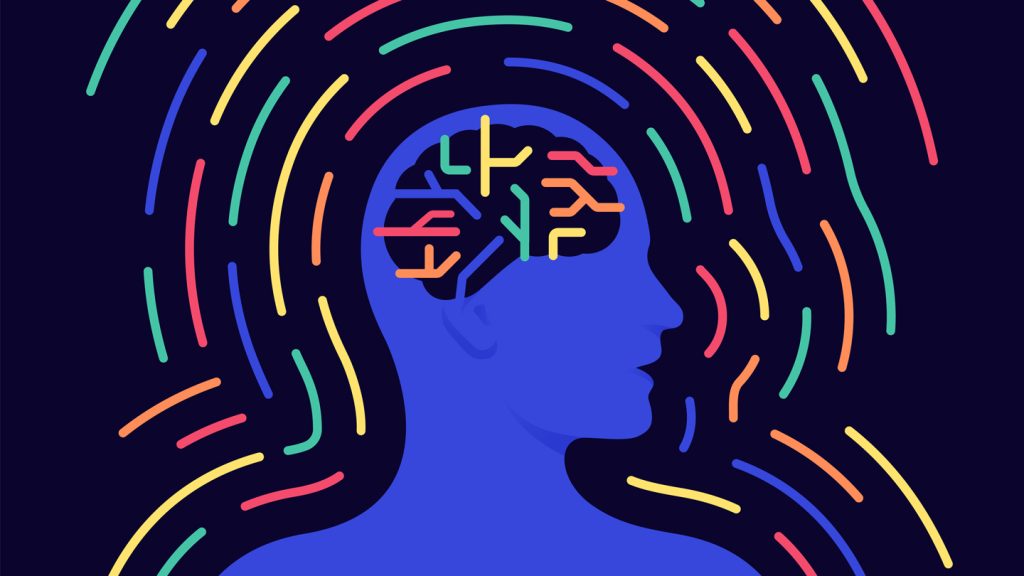
The classical view of how the human brain controls voluntary movement might not tell the whole story.
That map of the primary motor cortex — the motor homunculus — shows how this brain region is divided into sections assigned to each body part that can be controlled voluntarily (SN: 6/16/15). It puts your toes next to your ankle, and your neck next to your thumb. The space each part takes up on the cortex is also proportional to how much control one has over that part. Each finger, for example, takes up more space than a whole thigh.
A new map reveals that in addition to having regions devoted to specific body parts, three newfound areas control integrative, whole-body actions. And representations of where specific body parts fall on this map are organized differently than previously thought, researchers report April 19 in Nature.
Research in monkeys had hinted at this. “There is a whole cohort of people who have known for 50 years that the homunculus isn’t quite right,” says Evan Gordon, a neuroscientist at Washington University School of Medicine in St. Louis. But ever since pioneering brain-mapping work by neurosurgeon Wilder Penfield starting in the 1930s, the homunculus has reigned supreme in neuroscience.
Gordon and his colleagues study synchronized activity and communication between different brain regions. They noticed some spots in the primary motor cortex were linked to unexpected areas involved in action control and pain perception. Because that didn’t fit with the homunculus map, they wrote it off as a result of imperfect data. “But we kept seeing it, and it kept bugging us,” Gordon says.
So the team gathered functional MRI data on volunteers as they performed various tasks.
Two participants completed simple movements like moving just their eyebrows or toes, as well as complex tasks like simultaneously rotating their wrist and moving their foot from side to side.
The fMRI data revealed which parts of the brain activated at the same time as each task was done, allowing the researchers to trace which regions were functionally connected to one another. Seven more participants were recorded while not doing any particular task in order to look at how brain areas communicate during rest.
Testing only a few participants, each for many hours, offers unique insights into neural connectivity, Gordon says. “When we collect this much data in individuals, we constantly start seeing things that people have never really noticed before.”
The team discovered that while the brain-body part connections vaguely follow the pattern discovered by Penfield, the primary motor cortex is organized into three distinct sections. Each represents different body regions: lower body, torso and arms, and head.
Within each of these sections, the outermost body part of that region is mapped to the center of that section. For example, the area of the primary motor cortex assigned to the lower body has the toes in the middle with other leg parts radiating out in each direction from it. As a result, the entire section is organized like this: hip, knee, ankle, toes, ankle, knee, hip.
The team also unexpectedly found three mysterious spots not linked to a specific body part. Dubbed intereffector regions, they connect to an external network involved in action control and the sensing of pain. These regions alternate with the sections devoted to specific body parts. The team suspects that intereffector regions may integrate action goals and body movements involving multiple body parts, while the spaces in between are used for precise movements of isolated body parts.
Using previous data from three large fMRI studies, which include data from around 50,000 people, the team verified that this organization was consistent across a wide swath of people. Similar patterns also appeared in existing datasets from macaque monkeys, children and clinical populations.
“I think it was just easy to miss anything that seemed anomalous — must be noise,” says Michael Graziano, a neuroscientist at Princeton University who was not involved in the research. But with access to these huge datasets, “you get these vast numbers of subjects, and the pattern is crystal clear, and you can’t ignore it …. This is really the best example I’ve seen in a long, long time of looking at humans and trying to figure out at a detailed level what is the organization.”
Gordon’s team now plans to see whether these intereffector regions play a role in certain kinds of pain. More broadly, the team hopes their findings will prompt more in-depth research of what specific areas of the brain do. With new techniques and equipment, there is much left to explore, Gordon says. “Brain mapping isn’t dead.”