4 surprising things we just learned about Jupiter
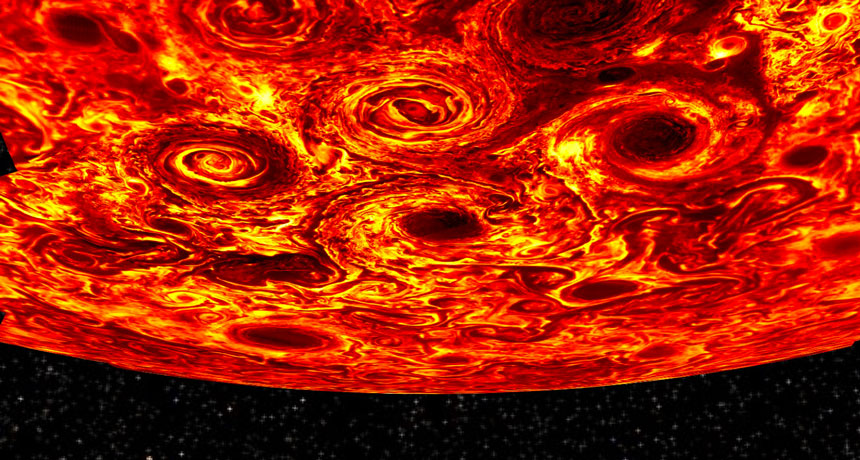
Bit by bit, Jupiter is revealing its deepest, darkest secrets.
The latest findings are in from the Juno spacecraft. And they unveil the roots of the planet’s storms, what lies beneath the opaque atmosphere and a striking geometric layout of cyclones parked around the gas giant’s north and south poles.
“We’re at the beginning of dissecting Jupiter,” says Juno mission leader Scott Bolton of the Southwest Research Institute in San Antonio. And the picture that’s emerging — still just a sketch — topples many preconceived notions. The results appear in four papers in the March 8 Nature.
Juno has been orbiting Jupiter since July 4, 2016, on a mission to map the planet’s interior (SN: 6/25/16, p. 16). The probe loops around once every 53 days, traveling on an elongated orbit that takes the spacecraft from pole to pole and as close as about 4,000 kilometers above the cloud tops.
As it plows through Jupiter’s gravity field, Juno speeds up and slows down in response to shifting masses inside the planet. By measuring these minute accelerations and decelerations, scientists can calculate subtle variations in Jupiter’s gravity and deduce how its mass is distributed. That lets researchers build up a three-dimensional map of the planet’s internal structure. At the same time, Juno snaps pictures in visible and infrared light. While other probes have extensively photographed much of the planet, Juno is the first to get an intimate look at the north and south poles.
“The whole thing is really intriguing, especially when you compare [Jupiter] to other giant planets,” says Imke de Pater, a planetary scientist at the University of California, Berkeley. “They are all unique, it looks like.”
Check out these four surprising new things we’ve learned that make Jupiter one of a kind:
- Rings of cyclones
Parked at each pole is a cyclone several thousand kilometers wide. That part isn’t surprising. But each of those cyclones is encircled by a polygonal arrangement of similarly sized storms — eight in the north and five in the south. The patterns have persisted throughout Juno’s visit.
“We don’t really understand why that would happen, and why they would collect up there in such a geometric fashion,” Bolton says. “That’s pretty amazing that nature is capable of something like that.”
- More than skin deep
Researchers have long debated whether the photogenic bands of clouds that wrap around Jupiter have deep roots or just skim the top of the atmosphere. Juno’s new look shows that the bands penetrate roughly 3,000 kilometers below the cloud tops. That’s 30 times as thick as the bulk of Earth’s atmosphere. While just a tiny fraction of Jupiter’s diameter, that’s deeper than previously thought, Bolton says. - Weighty weather
Within those 3,000 kilometers lies what passes for an atmosphere on Jupiter. It’s the stage on which Jupiter’s turbulent weather plays out. The atmosphere alone is about three times as massive as our planet, or 1 percent of Jupiter’s entire mass, researchers estimate. - Stuck together
Below the atmosphere, Jupiter is fluid. But unlike most fluids, the planet rotates as if it’s a solid mass. Like kids playing crack-the-whip, atoms of hydrogen and helium figuratively link arms and spin around the planet in unison, scientists report. Earlier results from Juno also indicate there’s no solid core lurking beneath this fluid (SN: 6/24/17, p. 14), so anyone dropped into the planet can expect a terribly long fall.
Many of these results are preliminary, and it’s unclear what it all means for how Jupiter operates. But what’s been learned so far, Bolton says, “is quite different than anybody anticipated.”